Our Team
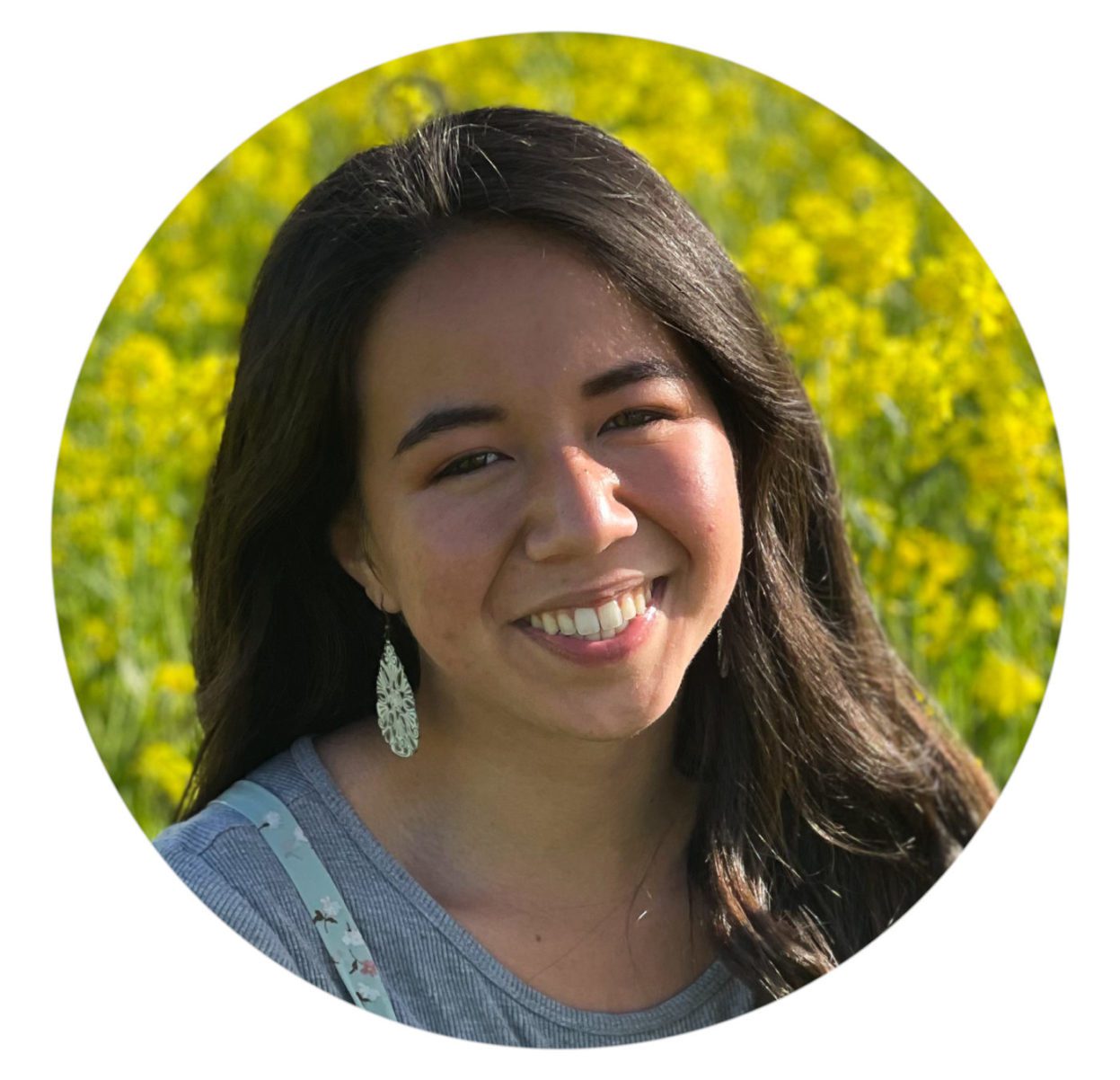
Bree Yang
4th year biomedical engineering student
Bree is a 4th year biomedical engineering student from Carlsbad, California. She has a strong interest in regenerative medicine and biomaterials, and she is considering pursuing an MS in Biomedical Engineering at Cal Poly. In her free time, she loves running, baking, and hiking in SLO with friends.
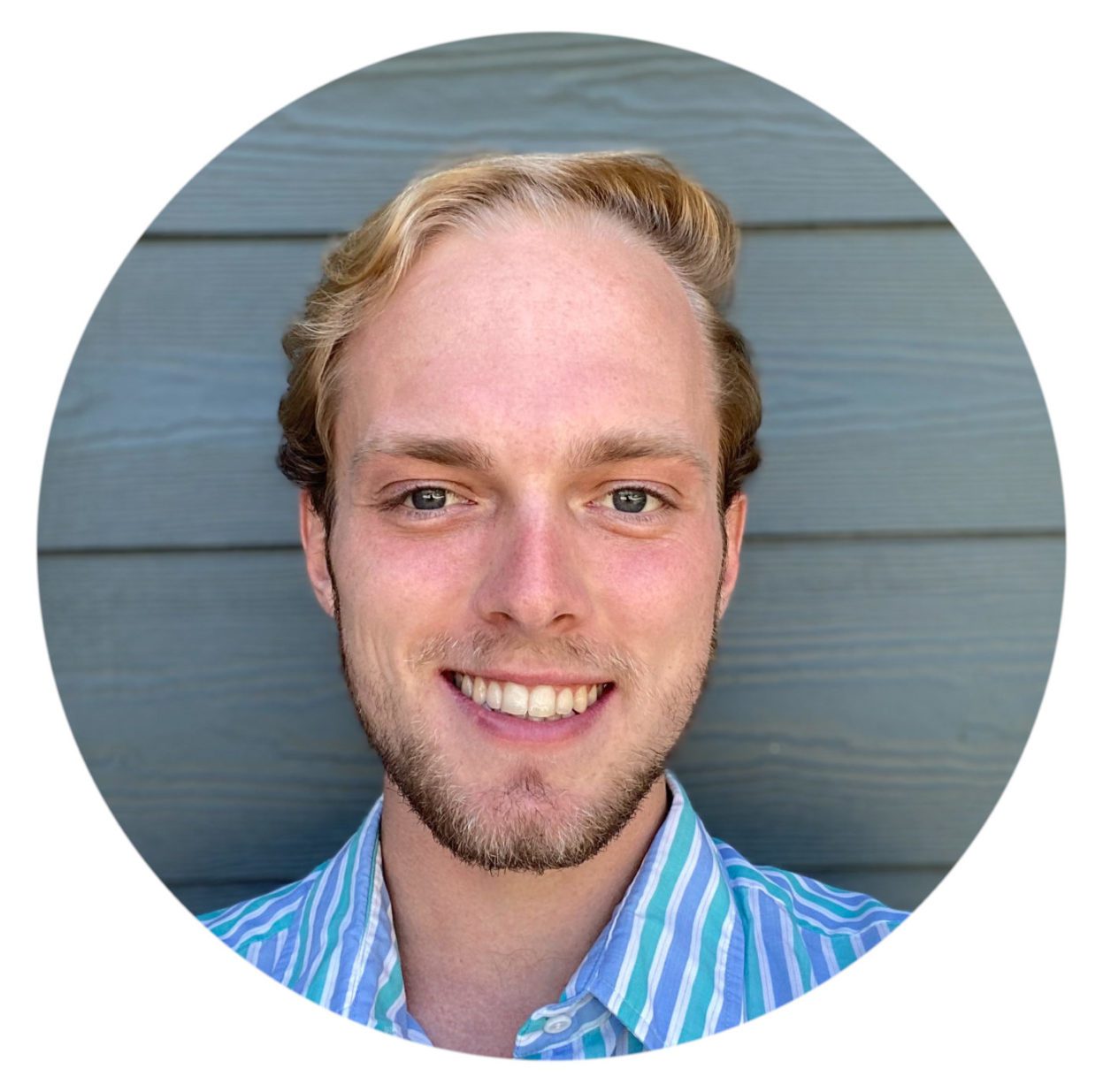
Jack Helmering
4th year biomedical engineering student
Jack is a 4th year biomedical engineering major from Vail, Colorado. As an aspiring biomedical engineer, Jack has spent his time at Cal Poly enhancing his knowledge of materials science and mechanical design through class, club, and project experiences. Outside of school, he enjoys making music, cooking, and spending time at Avila Beach.
If you have questions about the lab or would like to collaborate, please reach out to our advisor, Dr. Kristen Cardinal, at kohallor@calpoly.edu.
Acknowledgements
Special thanks to David Laiho, Squeaky Buentipo, and Eunice Alvarado for their assistance and support. We would also like to thank the Cal Poly Tissue Engineering Lab that made our research possible.
Our Project Video
Our Project's Digital Poster
INTRODUCTION
- Tissue-engineered in vitro “blood vessel mimics” (BVMs) are utilized to examine the endothelial cell response to intravascular devices [1]. They can be created by depositing endothelial cells on a cylindrical, biocompatible polymer scaffold [2].
- Scaffolds are fabricated through a technique called electrospinning. Electrospinning uses an electric field to create fibers from an extruded polymer-solvent solution [3]. Specific polymer-solvent solutions and electrospinning parameters refine scaffold properties [4].
- Polycaprolactone (PCL) is a flexible, malleable, and affordable polymer compared to other electrospinnable polymers, making it desirable for BVM scaffolds [5]. Currently, Cal Poly’s tissue engineering lab lacks a refined PCL electrospinning procedure [6].
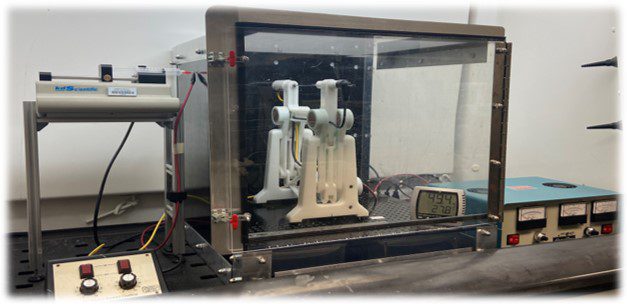
Figure 1. Electrospinning system at Cal Poly with syringe pump (left), electrospinning chamber (center), and voltage source (right).
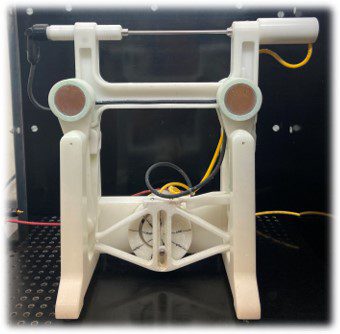
Figure 2. Rotating and translating mandrel collector for fiber collection.
OBJECTIVES
Overall Goal: Develop and implement a PCL scaffold fabrication procedure that produces consistent, desirable results.
1.Using literature, identify suitable solution parameters for electrospinning PCL.
2.Conduct trials with varying parameters to optimize outcomes with PCL.
3.Examine effectiveness of optimized PCL parameters.
METHODS
1.Literature Review of PCL Electrospinning
- Determined two feasible solvent systems: (1) dichloromethane (DCM) & dimethylformamide (DMF) and (2) tetrahydrofuran (THF) & DMF
- Reviewed effects of environmental, solvent, and processing parameters on electrospinning PCL
2. Electrospinning Trials
Solution Mixing -> Electrospinning -> Scaffold Desiccation
- Solvent and processing parameters were varied throughout trials to optimize specifications (see Table I)
- Temperature and humidity were monitored but not controlled (~50% – 60% RH and 20°C – 23°C)

3. Scaffold Evaluation
SEM Imaging -> Fiber Analysis -> Bioreactor Testing
- Imaged samples with Hitachi TM-1000 Tabletop SEM
- Analyzed fiber diameters with MATLAB-based SIMPoly image analysis method [7]
- Tested scaffold compatibility and durability with short-term bioreactor use
RESULTS
PCL with DCM & DMF (39 Trials)
Trial #5

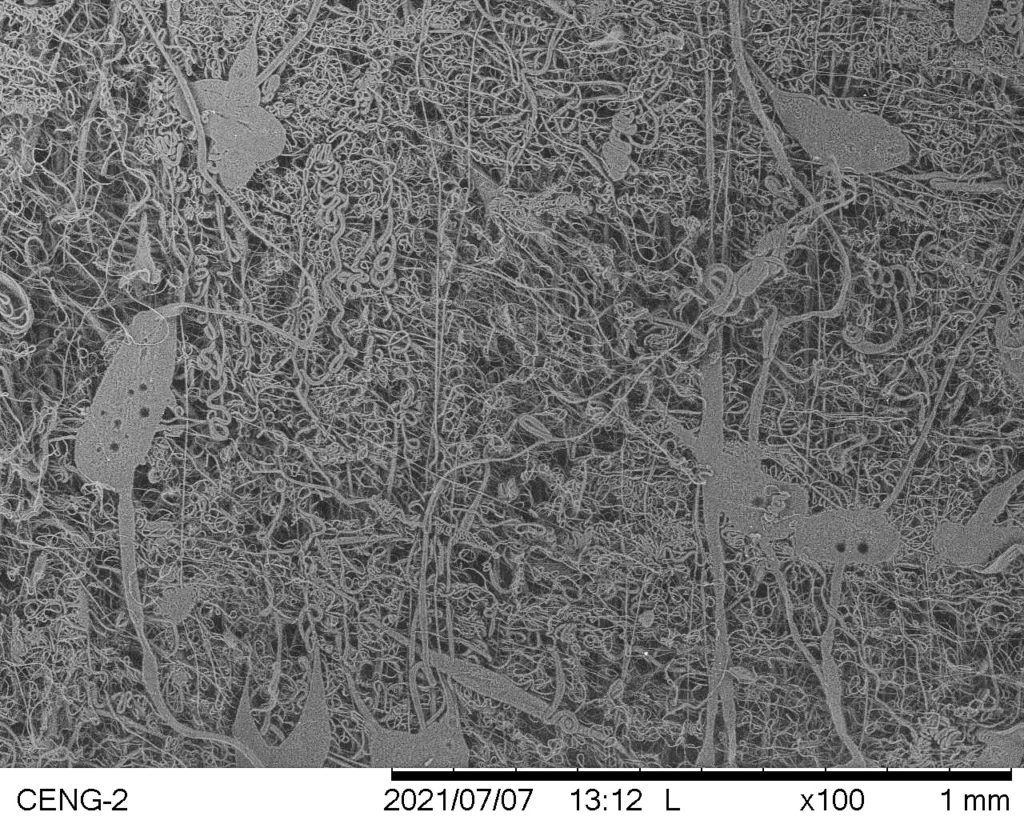
- Sparse, uneven fiber collection with significant solution dripping
- Large, irregular polymer masses among fibers
Avg. Fiber Diameter: 3.4276 µm
Standard Deviation: 0.7639 µm
Trial #14

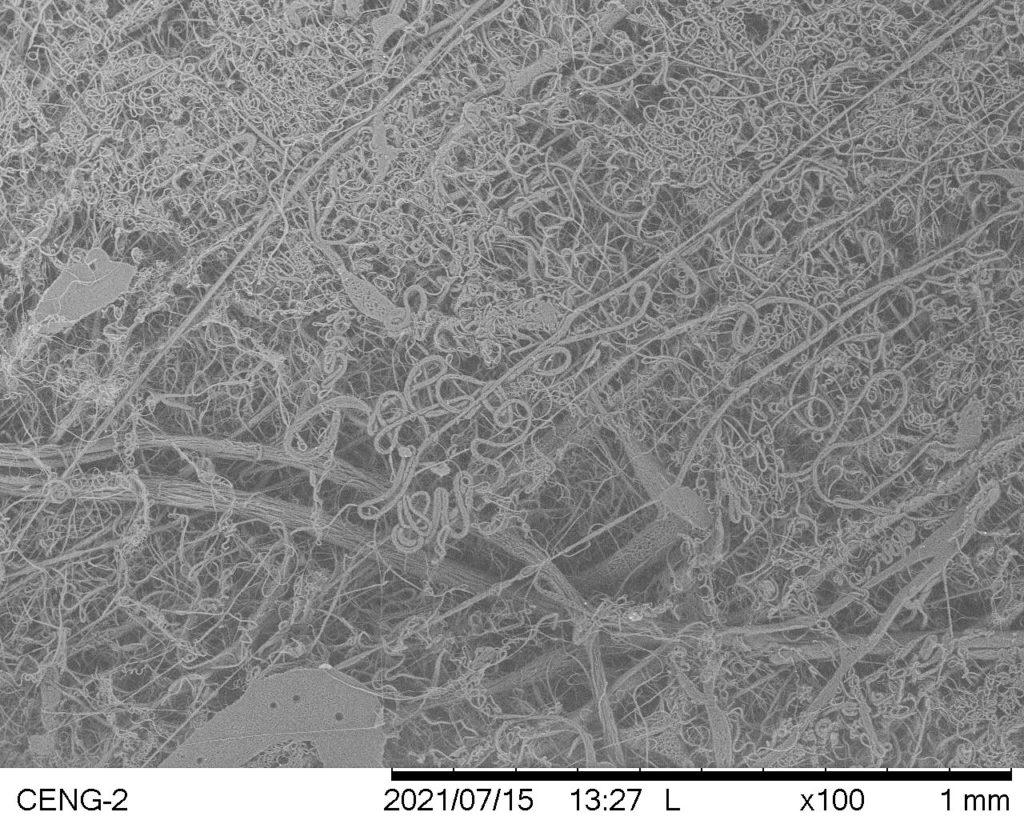
- Irregularly distributed fibers with uneven texture
- Large, irregular polymer masses among fibers
Avg. Fiber Diameter: 1.3979 µm
Standard Deviation: 0.3883 µm
Trial #15

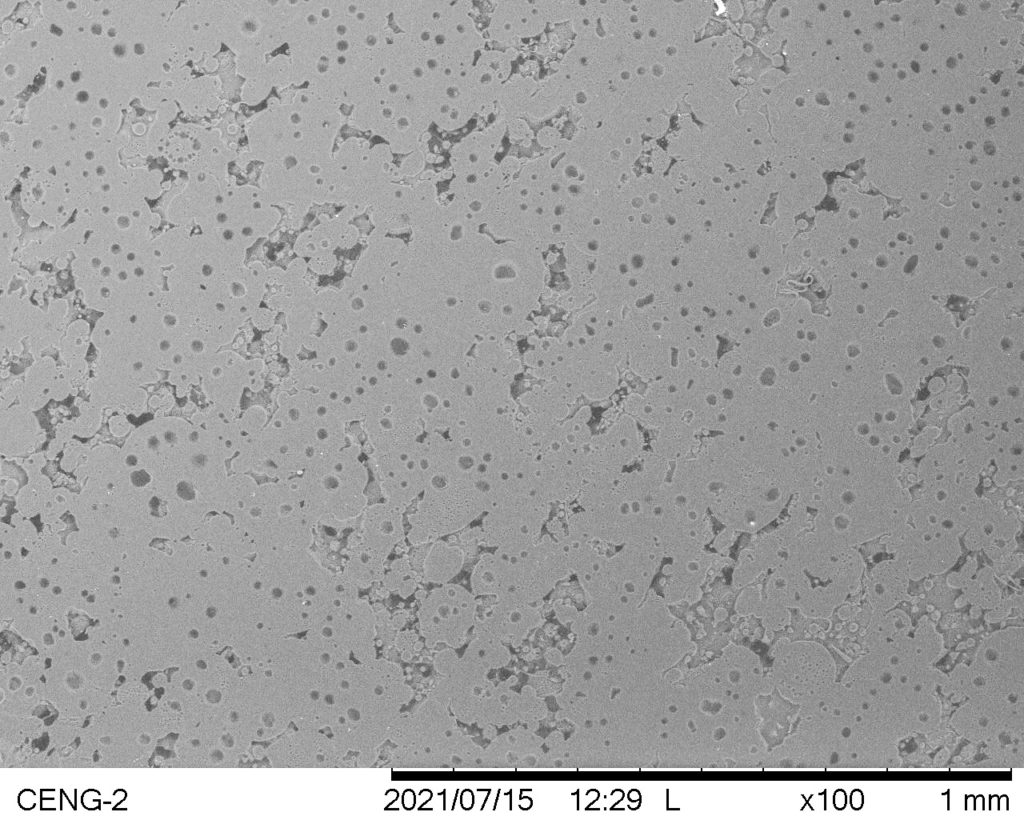
- Thin, smooth polymer collection with dripping
- No fiber formation; dense polymer sputtering on mandrel
Avg. Fiber Diameter: N/A
Standard Deviation: N/A
Trial #37

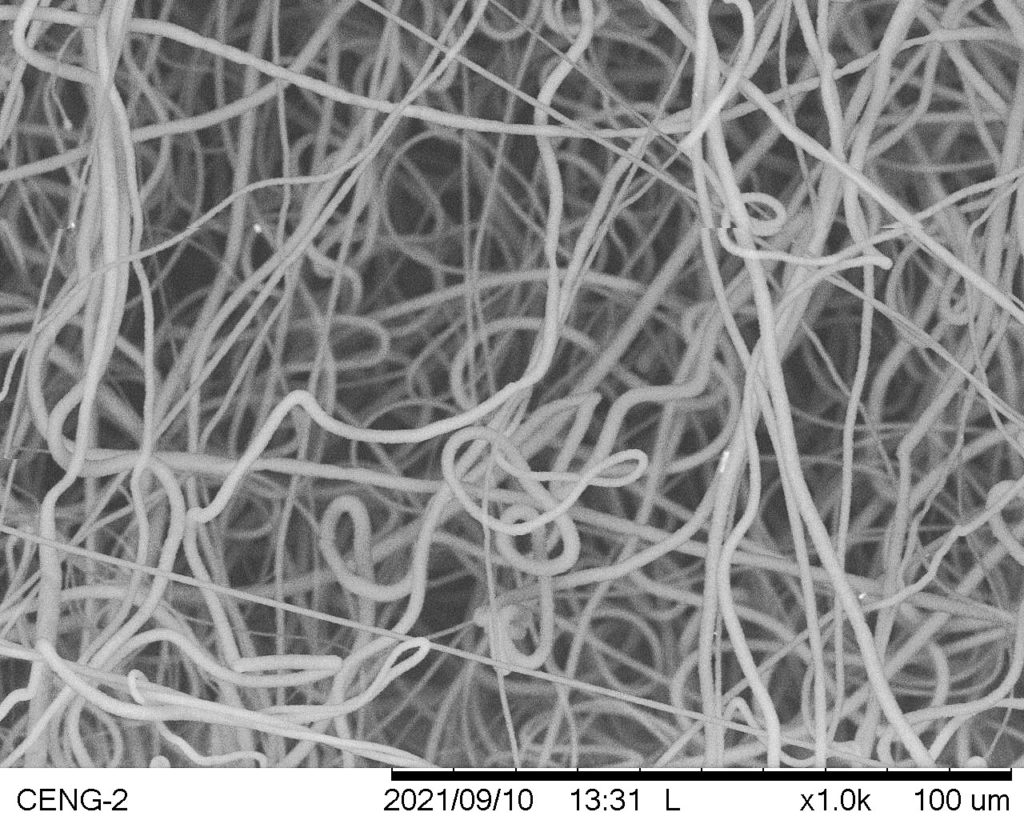
- Evenly distributed, thick fiber collection across mandrel with no dripping
- Small, uniform fibers with minimal clumping
Avg. Fiber Diameter: 1.8063 µm
Standard Deviation: 0.3531 µm
PCL with THF & DMF (41 Trials)
Trial #12

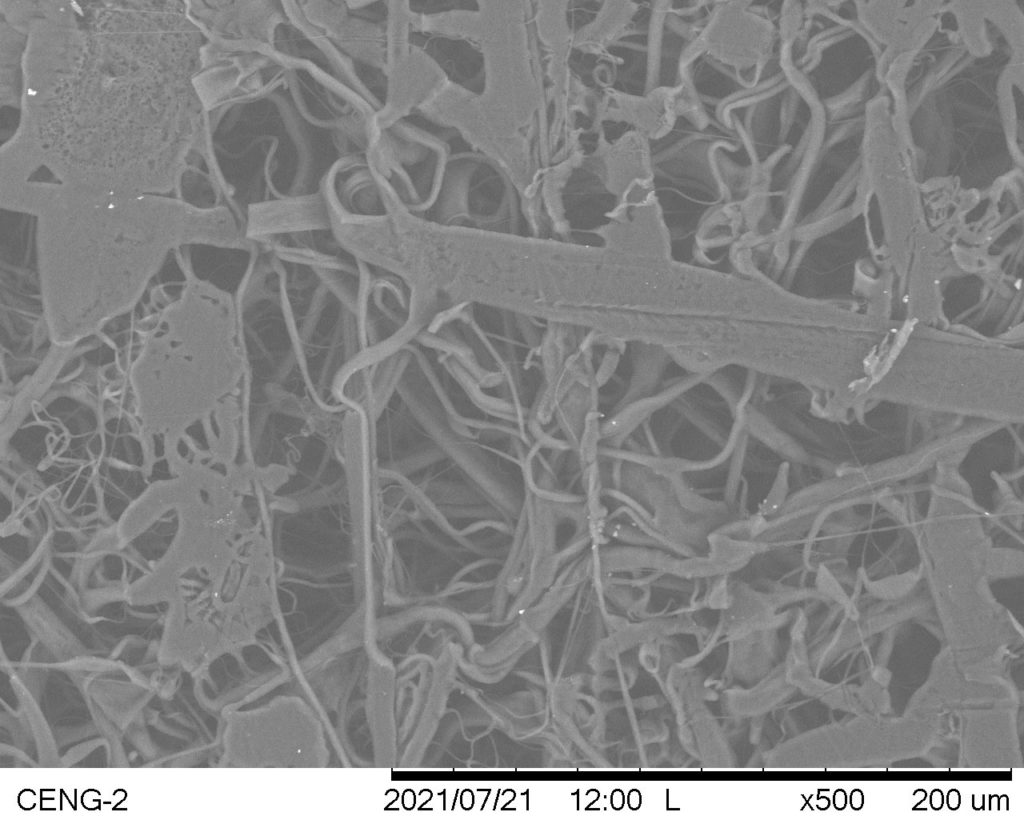
- Sparse, uneven fiber collection with significant dripping
- Large, irregular polymer masses among fibers
Avg. Fiber Diameter: N/A
Standard Deviation: N/A
Trial #18

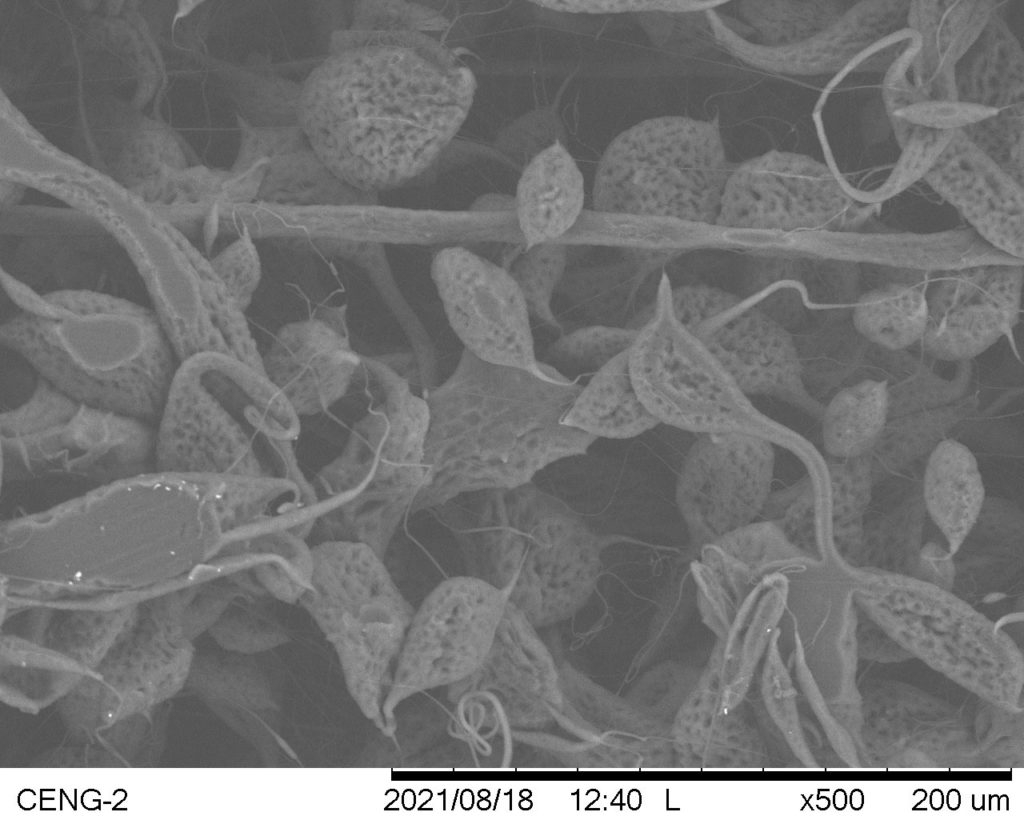
- Smooth, evenly distributed fibers with some bumps
- Porous beads, polymer clumps, and thick fibers
Avg. Fiber Diameter: N/A
Standard Deviation: N/A
Trial #26

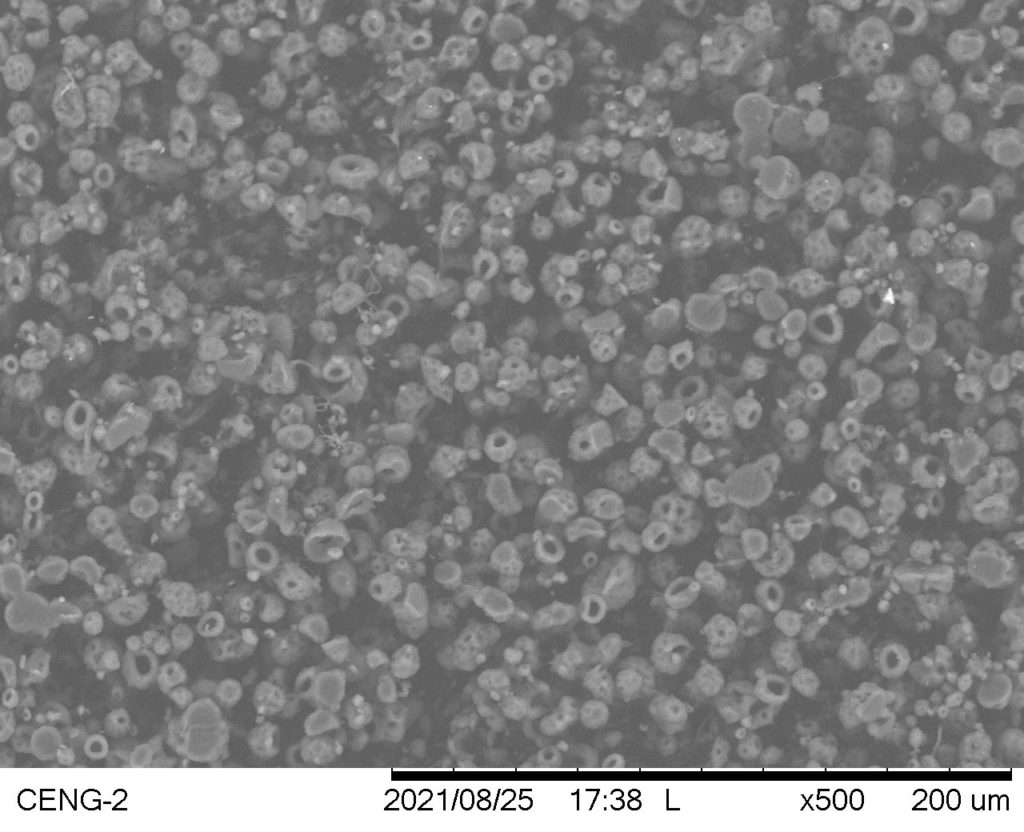
- Smooth, evenly distributed mandrel coverage
- No fiber formation; occurrence of electrospraying
Avg. Fiber Diameter: N/A
Standard Deviation: N/A
Trial #35

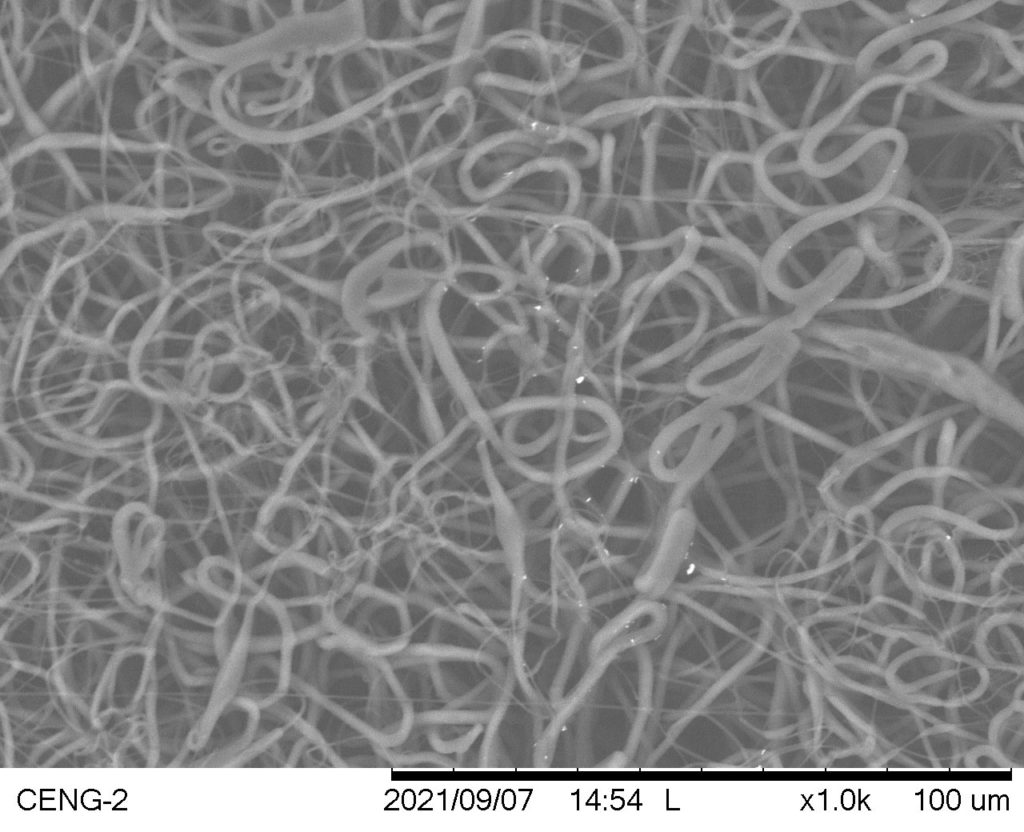
- Smooth, evenly distributed fiber collection with bumps across surface
- Small, mostly uniform fibers with minimal clumping and random distribution
Avg. Fiber Diameter: 1.9421 µm
Standard Deviation: 0.3825 µm
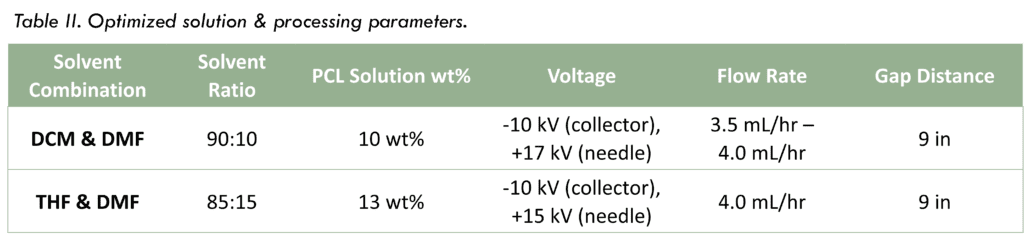
Bioreactor Evaluation
- Flexibility of each PCL scaffold allowed for easy attachment to bioreactor fittings.
- Each scaffold demonstrated it was capable of transmural flow, indicating cell media can perfuse in the system.
- Each scaffold sustained 1 hour of cell media flow via a peristaltic pump.
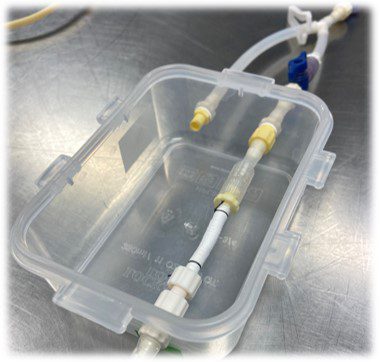
Figure 3. PCL scaffold with THF & DMF attached to fittings in bioreactor chamber.
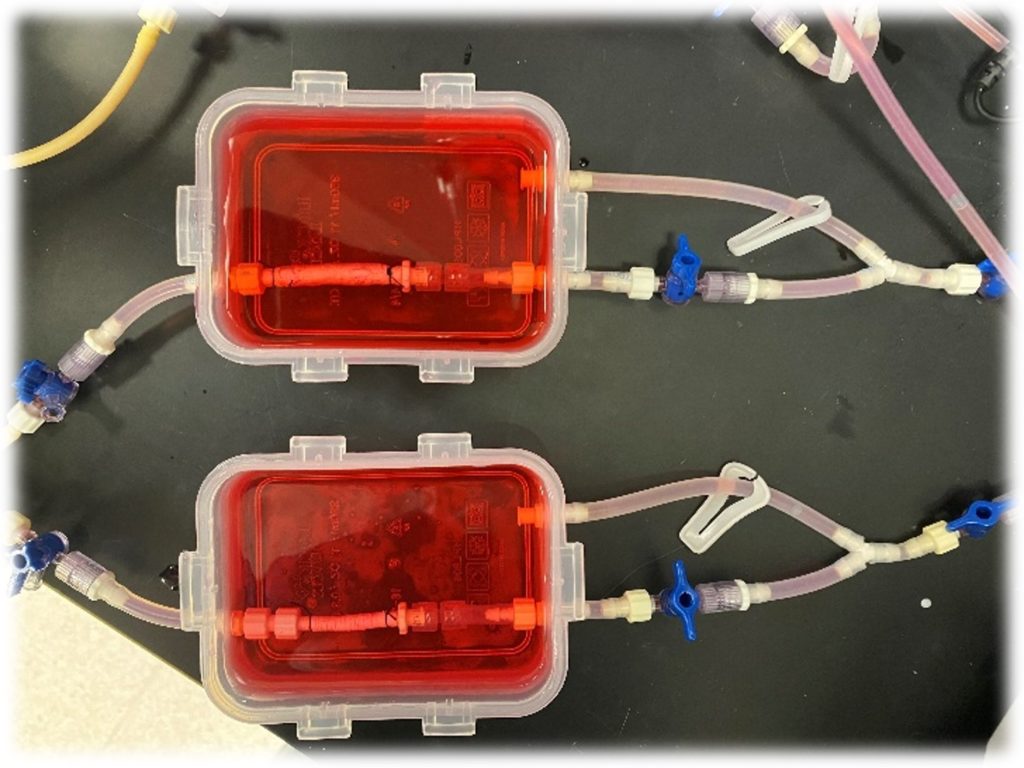
Figure 4. PCL scaffolds with THF & DMF (top) and DCM & DMF (bottom) in bioreactors with cell media flow.
DISCUSSION
- Low PCL wt% solutions (<10 wt% for DCM & DMF; <12 wt% for THF & DMF) were unable to produce fibers and resulted in beading or splattering.
- High PCL wt% solutions with DCM & DMF (<12 wt%) were too viscous to produce thin, uniform fibers.
- A 90:10 ratio of DCM:DMF resulted in smaller, more uniform fibers than a PCL solution with only DCM.
- Without the addition of DMF, a solution of only PCL and THF did not produce fibers.
- A chamber free of polymer residue and isolated from the external environment drastically improved results for both solvent systems.
Limitations include:
- The inability to precisely control temperature and humidity, which may have affected scaffold reproducibility.
- The narrow range of solvent ratios attempted during electrospinning trials.
FUTURE DIRECTIONS
- Perform mechanical testing, such as tensile testing, to quantify the mechanical properties of PCL scaffolds.
- Test biocompatibility of both optimized PCL scaffolds.
- Determine the rate and amount of endothelial cell adhesion to PCL scaffolds with both DCM & DMF and THF & DMF solvent systems.
- Conduct prolonged bioreactor setups with PCL scaffolds to analyze long-term scaffold durability and degradability.
- Implement mechanisms to control temperature and humidity within the chamber to examine environmental effects on scaffold results.
CONCLUSIONS
- A clean, isolated chamber is essential to producing consistent results.
- Both DCM & DMF and THF & DMF solvent systems can successfully produce PCL scaffolds with optimized electrospinning parameters.
- The solution wt% and solvent ratios should be tailored to each polymer-solvent combination.
ACKNOWLEDGEMENTS
Special thanks to David Laiho, Squeaky Buentipo, and Eunice Alvarado for their assistance and support. We would also like to thank Cal Poly’s Tissue Engineering Lab that made our research possible.
REFERENCES
1.Cardinal KOH, Williams SK. Assessment of the intimal response to a protein-modified stent in a tissue-engineered blood vessel mimic. Tissue Engineering Part A 15(12): 3869–3876, 2009.
2.Cardinal KOH, Bonnema GT, Hofer H, Barton JK, Williams SK. Tissue-engineered vascular grafts as in vitro blood vessel mimics for the evaluation of endothelialization of intravascular devices. Tissue Engineering 12(12): 3431–3438, 2006.
3.Jun I, Han HS, Edwards JR, Jeon H. Electrospun fibrous scaffolds for tissue engineering: Viewpoints on architecture and fabrication. International Journal of Molecular Sciences 19(3): 745, 2018.
4.Beachley V, Wen X. Effect of electrospinning parameters on the nanofiber diameter and length. Materials Science and Engineering: C 29(3): 663–668, 2009.
5.Nair N, Sekhar V, Nampoothiri K, Pandey A. Biodegradation of biopolymers. Current Developments in Biotechnology and Bioengineering: 739–755, 2017.
6.Youngblood A, Yakligian E, Cardinal KOH. Evaluation of new polymers for electrospinning tissue engineered blood vessel scaffolds. 2019 Summer Undergraduate Research Program Symposium, 2019.
7.Murphy R, Turcott A, Banuelos L, Dowey E, Goodwin B, Cardinal KOH. SIMPoly: A MATLAB-based image analysis tool to measure electrospun polymer scaffold fiber diameter. Tissue Engineering Part C: Methods 26(12): 628–636, 2020.